Thermophotovoltaic Efficiency Record May Lead to Compact Power
Thermophotovoltaic Efficiency Record May Lead to Compact Power
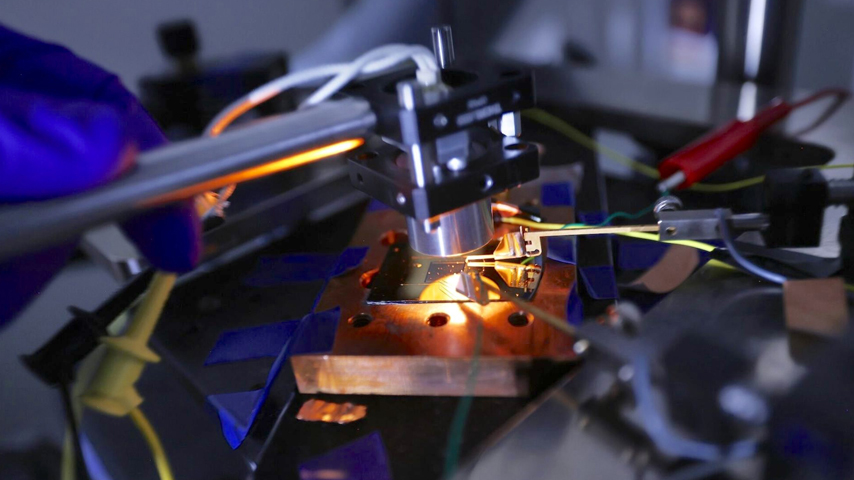
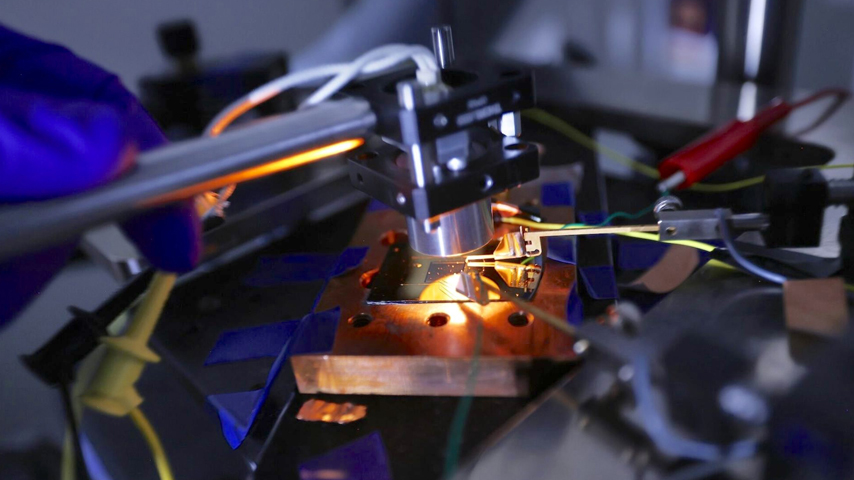
Improved solar cell converts 44 percent of infrared light to electricity. The breakthrough could advance energy storage and nuclear power.
Photovoltaic cells are an increasingly common sight, set up on fields and rooftops to convert visible light into electricity. While photovoltaics is a fairly mature technology, a related technology has begun to receive more research attention. Thermophotovoltaic cells rely on longer-wavelength, lower-energy infrared photons rather than visible light to produce an electric current.
The promise of thermophotovoltaic (TPV) cells is that they could scavenge waste heat radiated from exhaust pipes or stored over long periods in hot solid material. As part of a heat battery, these cells could be connected to the grid to store intermittent renewable energy during peak production hours. But because the temperature of these hot sources is far less than that of the sun, the efficiency of TPV cells has lagged that of conventional photovoltaics.
A recent breakthrough has improved the efficiency of these cells considerably, to the point where they are closing in on their theoretical limit. According to researchers, an array of these high-efficiency TPV cells about the size of a living room could produce 1 MW of electricity.
Andrej Lenert, associate professor of chemical engineering at the University of Michigan, has been working to increase the efficiency of TPV cells so they can provide viable energy storage in the power grid. This required building a prototype cell to retrieve the energy stored inside a heat battery, where the thermophotovoltaics surround a block of heated material at a temperature of at least 1,000 °C.
“Essentially, using electricity to heat something up is a very simple and inexpensive method to store energy, compared to lithium-ion batteries,” Lenert said. “It also gives you access to many different materials to use as a storage medium for thermal batteries.”
The heated storage material radiates thermal photons with a range of energies. At 1,435 °C, about 20 to 30 percent of those have enough energy to generate electricity in the team’s thermophotovoltaic cells.
“Our goal was to optimize the semiconductor material, which captures the photons, to broaden its preferred photon energies while aligning with the dominant energies produced by the heat source,” Lenert said.
Read the Energy Blog: Heat Batteries Step into the Spotlight
Lenert began looking at the challenge of improving TPV efficiencies more than a decade ago, when he was a doctoral student at MIT. But he started off working on a different part of the heat storage problem.
“My focus had been on changing the spectrum of the heat source, which is very difficult to do at high temperatures,” he said. “Late in my PhD, I realized that it should be possible to re-design solar cells to work as very efficient optical filters, absorbing only short wavelengths and reflecting the rest.”
To capture photons above and below the energies that the semiconductor can convert to electricity, the researchers built a thin layer of air into the thermophotovoltaic cell just beyond the semiconductor. They then added a gold reflector beyond the air gap—a structure called an air bridge. This cavity helps trap photons with the right energies so that they entered the semiconductor and sent the rest back into the heat storage material, where the energy had another chance to be re-emitted as a photon the semiconductor could capture.
The strategy worked—the prototype thermophotovoltaic cell delivered a power conversion efficiency of 44 percent at 1,435 °C, within the target range for existing high-temperature energy storage (1,200 °C to 1600 °C). It surpasses the previous record 37 percent achieved by previous designs within this temperature range.
More on This Topic: 6 Ways Solar-Powered Technology Blends into Everyday Life
Lenert believes that the heat recovery efficiency limit for this type of battery could reach 50 percent.
“The novelty of the air-bridge design is that it reflects nearly all of those ‘low-energy’ photons back to the heat source,” he added, “giving them another chance of being emitted as useful, high-energy photons.”
Air-bridge thermophotovoltaic cells are fabricated in a range of band gaps from 0.74 eV to 1.1 eV. Cells reflect nearly all the incident below-band-gap radiation. Air-bridge thermophotovoltaic cells have demonstrated enhanced power conversion efficiencies by recuperating a large amount of power carried by below-band-gap (out-of-band) photons.
Based on Your Interests: Green Power Employment Outlook
“Our results,” Lenert said, “suggest the applicability of the air-bridge cells to a range of semiconductor systems suitable for electricity generation from thermal sources found in both consumer and industrial applications, including thermal batteries.”
Unlike solar cells, thermophotovoltaic cells are placed right next to the high-temperature heat source. This allows them to theoretically produce 50 to 100 kW per square meter, about 500 times that of rooftop solar. The research team’s next aim is to demonstrate that power density while reaching 50 percent efficiency.
“With such performance, we can envision building modular power systems that use only 20 m2 of our cells to produce 1 MW of electricity, enough to power a small town,” Lenert said.
Outside of energy storage, this breakthrough may have significant impacts in nuclear energy, particularly in conjunction with small modular reactors, and in space technologies.
Mark Crawford is a technology writer in Corrales, N.M.
The promise of thermophotovoltaic (TPV) cells is that they could scavenge waste heat radiated from exhaust pipes or stored over long periods in hot solid material. As part of a heat battery, these cells could be connected to the grid to store intermittent renewable energy during peak production hours. But because the temperature of these hot sources is far less than that of the sun, the efficiency of TPV cells has lagged that of conventional photovoltaics.
A recent breakthrough has improved the efficiency of these cells considerably, to the point where they are closing in on their theoretical limit. According to researchers, an array of these high-efficiency TPV cells about the size of a living room could produce 1 MW of electricity.
Andrej Lenert, associate professor of chemical engineering at the University of Michigan, has been working to increase the efficiency of TPV cells so they can provide viable energy storage in the power grid. This required building a prototype cell to retrieve the energy stored inside a heat battery, where the thermophotovoltaics surround a block of heated material at a temperature of at least 1,000 °C.
“Essentially, using electricity to heat something up is a very simple and inexpensive method to store energy, compared to lithium-ion batteries,” Lenert said. “It also gives you access to many different materials to use as a storage medium for thermal batteries.”
The heated storage material radiates thermal photons with a range of energies. At 1,435 °C, about 20 to 30 percent of those have enough energy to generate electricity in the team’s thermophotovoltaic cells.
“Our goal was to optimize the semiconductor material, which captures the photons, to broaden its preferred photon energies while aligning with the dominant energies produced by the heat source,” Lenert said.
Read the Energy Blog: Heat Batteries Step into the Spotlight
Lenert began looking at the challenge of improving TPV efficiencies more than a decade ago, when he was a doctoral student at MIT. But he started off working on a different part of the heat storage problem.
“My focus had been on changing the spectrum of the heat source, which is very difficult to do at high temperatures,” he said. “Late in my PhD, I realized that it should be possible to re-design solar cells to work as very efficient optical filters, absorbing only short wavelengths and reflecting the rest.”
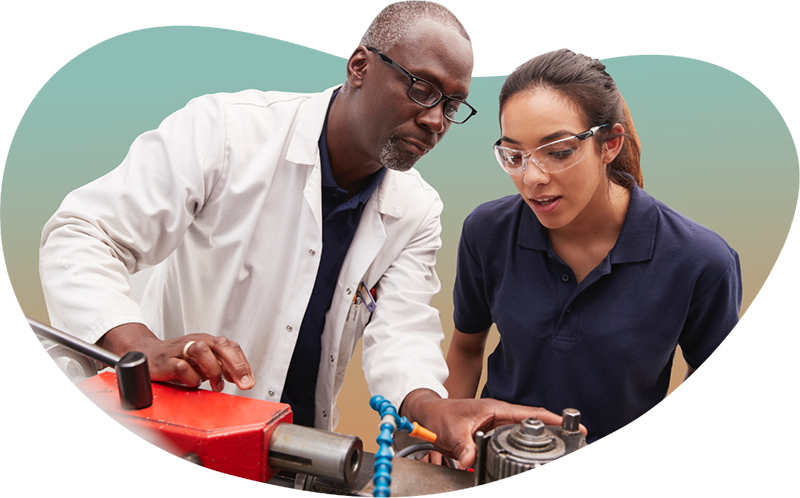
Join Our Community
ASME Membership provides you an instant community of engineers, opportunities to stay on top of industry trends, and a host of other valuable benefits.
The strategy worked—the prototype thermophotovoltaic cell delivered a power conversion efficiency of 44 percent at 1,435 °C, within the target range for existing high-temperature energy storage (1,200 °C to 1600 °C). It surpasses the previous record 37 percent achieved by previous designs within this temperature range.
More on This Topic: 6 Ways Solar-Powered Technology Blends into Everyday Life
Lenert believes that the heat recovery efficiency limit for this type of battery could reach 50 percent.
Redesigned cell
In thermophotovoltaic cells, unlike in solar cells, more than 80 percent of the incident light cannot excite electrons and traditionally goes to waste.“The novelty of the air-bridge design is that it reflects nearly all of those ‘low-energy’ photons back to the heat source,” he added, “giving them another chance of being emitted as useful, high-energy photons.”
Air-bridge thermophotovoltaic cells are fabricated in a range of band gaps from 0.74 eV to 1.1 eV. Cells reflect nearly all the incident below-band-gap radiation. Air-bridge thermophotovoltaic cells have demonstrated enhanced power conversion efficiencies by recuperating a large amount of power carried by below-band-gap (out-of-band) photons.
Based on Your Interests: Green Power Employment Outlook
“Our results,” Lenert said, “suggest the applicability of the air-bridge cells to a range of semiconductor systems suitable for electricity generation from thermal sources found in both consumer and industrial applications, including thermal batteries.”
Unlike solar cells, thermophotovoltaic cells are placed right next to the high-temperature heat source. This allows them to theoretically produce 50 to 100 kW per square meter, about 500 times that of rooftop solar. The research team’s next aim is to demonstrate that power density while reaching 50 percent efficiency.
“With such performance, we can envision building modular power systems that use only 20 m2 of our cells to produce 1 MW of electricity, enough to power a small town,” Lenert said.
Outside of energy storage, this breakthrough may have significant impacts in nuclear energy, particularly in conjunction with small modular reactors, and in space technologies.
Mark Crawford is a technology writer in Corrales, N.M.